While “climate change” only refers to fluctuations in the climate that last for decades or longer, “climate variability” refers to all variations in the climate that last longer than individual weather occurrences. Although the word “climate change” can be applied to any period of Earth’s history, it is currently most frequently used to refer to modern climate change, sometimes known as “global warming.” Human activity has been influencing the climate more and more since the Industrial Revolution.[1]
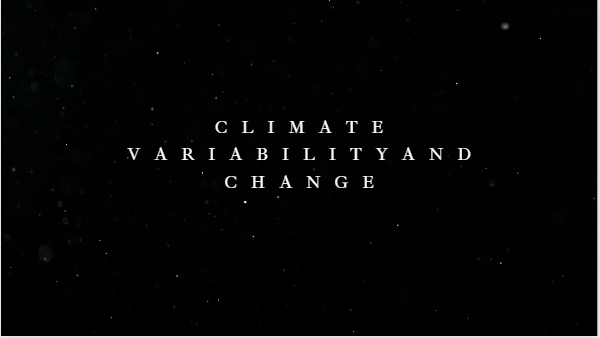
The sun provides almost all of the energy needed for the climate system, which then radiates that energy into space. Earth’s energy budget is the sum of energy entering the planet, leaving it, and moving through the climate system.
Earth’s energy budget is positive and the climate system is warming when entering energy exceeds outgoing energy. Earth cools and the energy budget becomes negative if more energy is lost.
The weather is a manifestation of the energy circulating through Earth’s climate system, which varies over time and across geographic scales. A region’s climate is defined by its long-term averages and weather variability. “Internal variability” refers to the natural processes that occur naturally in the different components of the climate system and modify the energy distribution. Ocean basin variability, such as the Pacific decadal oscillation and the Atlantic multidecadal oscillation, are two examples. External forcing, or events that occur outside of the components of the climate system, can also lead to variations in climatic variability.
Causes of Climate variability and change
The Earth’s equilibrium temperature and climate are determined, in the widest sense, by the rate at which energy is received from the Sun and the rate at which it is lost to space. Winds, ocean currents, and other mechanisms disperse this energy throughout the world, influencing the climates of various locations.[8]
“Forcing mechanisms” or “climate forcings” are variables that have the power to alter the climate.[9] These include phenomena like fluctuations in the Earth’s orbit, variations in solar radiation, variations in the albedo or reflectivity of the continents, atmosphere, and oceans, variations in the formation of mountains and continental drift, and variations in the concentrations of greenhouse gases. External forcing can be natural (such as variations in the Earth’s orbit or solar output) or man-made (such as increased emissions of greenhouse gases and dust).
Numerous feedbacks related to climate change have the potential to increase or decrease the initial forcing. Additionally, there are important benchmarks that, if crossed, can result in abrupt or permanent change.
In response to climate forcings, certain components of the climate system—like the oceans and ice caps—respond more slowly, while other components react more swiftly. The cooling of the atmosphere following a volcanic eruption, caused by sunlight reflected by volcanic ash, is an illustration of rapid change. After the atmosphere warms, ocean water expands thermally slowly over thousands of years. Combinations are also conceivable, such as the Arctic Ocean’s abrupt loss of albedo due to sea ice melting and the water’s more slow thermal expansion.
Internal variability
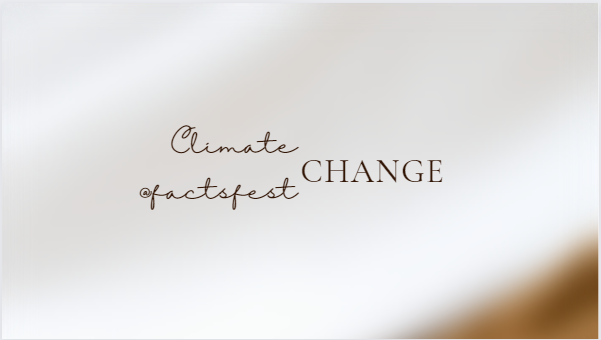
Internal variability-related climate changes can occasionally take the form of cycles or oscillations. Other forms of natural climate change are referred to be random or stochastic since we are unable to forecast when they will occur.[12] When it comes to climate, the weather might be seen as unpredictable.[13] There is an energy imbalance and the oceans may absorb more heat if there are few clouds in a given year.
This signal is’stored’ in the ocean and manifests as variability on time scales longer than the initial weather disturbances because of climate inertia.[14] The inertia of glaciers or oceans can convert weather disturbances that are entirely random and appear as white noise into climate shifts where longer-duration oscillations are also greater oscillations, a phenomenon called red noise.
There are cyclical and random components to many climate changes. We refer to this behavior as stochastic resonance.[15] Klaus Hasselmann and Syukuro Manabe shared half of the 2021 Nobel Prize in Physics for this study, which was related to their work on climate modeling. The other half went to Giorgio Parisi, who mostly received recognition for his theoretical physics work but also established the idea of stochastic resonance with collaborators [16].
Ocean-atmosphere variability
Together, the ocean and atmosphere have the capacity to produce internal climatic variability on their own, which can last for several years or even decades.[17][18] By redistributing heat between the deep ocean and the atmosphere, these fluctuations can have an impact on the average surface temperature of the entire planet.and/or by changing the distribution of clouds, water vapor, and sea ice, which might have an impact on the Earth’s overall energy balance.[21][22]
Oscillations and cycles
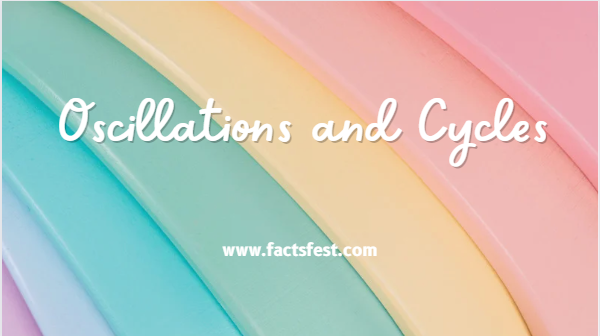
Any cyclical oscillation that occurs repeatedly within the global or regional climate is referred to as a climate oscillation or cycle. Since they are not precisely periodic—rather, they are quasiperiodic—a Fourier analysis of the data shows no clear peaks in the spectrum. Numerous oscillations on various time-scales have been observed or theorized:[23]
the Southern Oscillation of El Niño (ENSO) a widespread phenomenon in the Pacific Ocean that alternates between warmer (El Niño) and colder (La Niña) tropical sea surface temperatures. It is an oscillation that sustains itself, and its processes are widely understood.24]
The most well-known recognized cause of interannual variability in the weather and climate on a global scale is ENSO.
The cycle happens every two to seven years, and within the longer term cycle, El Niño lasts for nine months to two years.[25] As a result of increased upwelling of cold waters off the west coast of South America, the cold tongue of the equatorial Pacific Ocean is warming more slowly than the rest of the ocean.[26][27]
The Madden-Julian oscillation (MJO) is a 30- to 60-day phase of higher rainfall over the tropics that moves eastward and is primarily seen over the Pacific and Indian oceans.(28)
the North Atlantic oscillation (NAO): NAO indicators are determined by comparing the normalized sea-level pressure (SLP) differences between Stykkishólmur/Reykjavík, Iceland, and Ponta Delgada, Azores. Over the middle latitudes, stronger-than-average westerlies are indicated by positive values of the index.[29]
The well-understood oscillation in wind patterns in the stratosphere surrounding the equator is known as the quasi-biennial oscillation. The predominant wind shifts from easterly to westerly and back during a 28-month period.[30]
Some climate models forecast an oscillation in the climate called the Pacific Centennial Oscillation.
The predominant pattern of sea surface variability in the North Pacific on a decadal scale is known as the Pacific decadal oscillation.
A “warm” or “positive” phase causes the west Pacific to cool while warming portions of the eastern ocean; a “cool” or “negative” phase causes the reverse pattern to occur. It is believed to be a conglomeration of various physical processes rather than a singular entity.[31]
The Pacific Ocean’s basin-wide variability, known as the Interdecadal Pacific Oscillation (IPO), has a period of 20 to 30 years.[32]
The Atlantic multidecadal oscillation is a 55–70 year pattern of variability in the North Atlantic that affects rainfall, droughts, and the frequency and strength of hurricanes.[33]
Tens of thousands of years is the duration of the North African Monsoon, which drives climate fluctuation in the region.[34]
both the Antarctic (AAO) and Arctic (AO) oscillations The annular modes are naturally occurring patterns of climate variability that span the hemisphere. They account for 20–30% of the variability in their respective hemispheres during periods ranging from weeks to months. The Antarctic oscillation (AAO) in the southern hemisphere and the Arctic oscillation (AO) in the northern hemisphere are known as the Southern Annular Mode and Northern Annular Mode, respectively.
Because the annular modes change the average courses of storms, they have a significant impact on the temperature and precipitation of land masses in mid-to-high latitudes, such Europe and Australia. One may think of the NAO as an AO/NAM regional index.[35] The initial EOF of sea level pressure or geopotential height between 20°N and 90°N (NAM) or 20°S and 90°S (SAM) is what defines them.
Dansgaard-Oeschger cycles: these occurred during the Last Glacial Maximum in around 1,500-year cycles.
Ocean current changes
Because the ocean has hundreds of times more mass than the atmosphere and, therefore, very high thermal inertia, the oceanic aspects of climate variability can produce variability on century periods. For instance, changes to ocean circulation patterns, such as thermohaline circulation, are crucial for the global ocean’s heat distribution.
A significant amount of energy is moved by ocean currents from the warmer tropical regions to the colder polar regions. The last ice age, or more accurately, the last glacial period, is a great example of how abrupt and significant changes in the North Atlantic circulation may cause variations in the global climate, even in the face of relatively constant energy input into the system.
External climate forcing
Greenhouse gases
climatic scientists usually classify greenhouse gases emitted from volcanoes as external, although greenhouse gases released by the biosphere are often considered as a feedback or internal climatic mechanism.[50] By absorbing infrared light, greenhouse gases like CO2, methane, and nitrous oxide warm the climate system. Another component of the extended carbon cycle is volcanoes.
They offset the uptake by sedimentary rocks and other geological carbon dioxide sinks by releasing carbon dioxide from the Earth’s crust and mantle over very long (geological) time periods.
Since the start of the industrial revolution, humans have increased greenhouse gas emissions by burning fossil fuels, changing the way land is used through deforestation, and releasing trace gases (such as nitrogen oxides, carbon monoxide, and methane) into the atmosphere.[52] A number of other variables come into play as well, such as land usage, ozone depletion, animal husbandry (ruminant animals like cattle create methane[53]), and deforestation.[54]
According to US Geological Survey estimates, present human activities produce 100–300 times the amount of carbon dioxide emitted by volcanoes, meaning that volcanic emissions are substantially lower.[55] It’s possible that the yearly amount released by human activity exceeds the amount released by supereruptions.
Orbital variations
The seasonal distribution of sunlight reaching the Earth’s surface and its global dispersion are influenced by minute fluctuations in Earth’s motion. The annual average sunshine in a given place doesn’t vary much, but the distribution’s seasonal and geographic fluctuations can be quite significant. Variations in Earth’s eccentricity, shifts in the tilt angle of the axis of rotation, and precession of the axis are the three categories of kinematic change. When combined, these result in Milankovitch cycles that have an impact on climate.
These cycles are notable because they are correlated with glacial and interglacial eras, the advance and retreat of the Sahara, and their occurrence in the stratigraphic record.[58][59]
Temperatures and CO2 concentrations were highly correlated during the glacial periods. It has become evident that this isn’t always the case, despite early research suggesting that CO2 concentrations followed temperatures.[60] The solubility of CO2 diminishes with increasing ocean temperatures, causing it to escape the water. Other factors of climate change may also have an impact on the flow of CO2 between the air and the ocean.[61] Little variations in the Earth’s motion can have a significant impact on climate due to these and other self-reinforcing mechanisms.[60]